Introduction
Voltage-dependent Ca2+ (CaV) channels form an important route for Ca2+ entry into cells, upon deviations from the cell’s resting membrane potential. Functionally, CaV channels are divided into Low Voltage-Activated (LVA) and High Voltage-Activated (HVA) channels1.
This functional division implies that cells respond differentially to small or large depolarization of the plasma membrane, activating two different classes of CaV channels, which conduct the Ca2+ inflow with different characteristics. T-type currents are carried out via channel proteins encoded by three genes that compose a subfamily within the CaV channels family (see Table 1)2-4. Several biophysical properties distinguish LVA from HVA CaV channels. The “T” stands for transient, resulting from the strong inactivation (in both the time and voltage domains), which is one of the hallmarks of these currents2.
The activity of T-type channels contributes to several known physiological and pathophysiological phenomena including burst firing in neurons, pace making activity in the heart and secretion from endocrine tissues. There are three cloned T-type channel isoforms, CaV3.1 (α1G) and CaV3.2 (α1H) are widely distributed while CaV3.3 (α1I) expression is restricted to the Central Nervous System (CNS, see Table 1). T-type channels are also involved in the acrosome reaction in sperm cells and in contraction of smooth muscle. CaV3.2 and CaV3.1 are expressed in kidney, but little is known about the physiological role of these channels there.
Functional HVA channels are multimers composed of pore forming α1 and several auxiliary (α2-δ, β and γ) subunits. However, for LVA channels the issue of interaction with auxiliary subunits is not fully determined yet2.
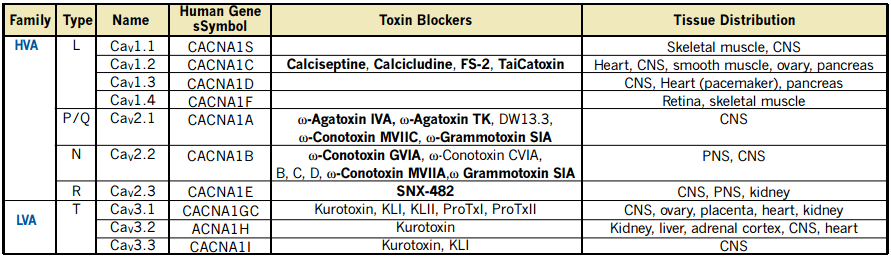
Pharmacology of T-Type Channels
Several compounds belonging to anesthetics, antipsychotics, antiepileptics and antihypertensives groups, which are in clinical use for different conditions, were found to affect the activity of T-type channels. As such, none of these compounds is a selective blocker of T-type channels, although some of these are effective inhibitors or block differentially the three T-type isoforms (enabling the pharmacological isolation of T-type components).
In addition, the T-type blocking activity of several of these compounds is within the clinical doses, implying that their therapeutic activity also involves the block of CaV LVA currents2. One example of a D2 dopamine receptor antagonist is Pimozide, which is used as an antipsychotic drug and was shown recently to block cloned CaV3 channels. In HEK cells expressing the 3 types of cloned T-type channels Pimozide inhibited the CaV3.1, 3.2 and 3.3 currents with Kd values of 43, 58 and 39nM respectively5. In Xenopus oocytes expressing CaV3.1 channels, Pimozide was less potent, with an IC50 of about 8mM (see Figure 1).
For more information, please refer to the List of Products, page 36, table 56. Dihydropyridins, which are considered as selective L-type channel modulators, were shown to affect LVA and T-type channels as well2.
Peptidyl Toxins that Block T-Type Channels
Kurotoxin was the first peptidyl toxin (isolated from the scorpion Parabuthus transvaalicus) that was shown to block specifically T-type channels6, although in native neurons it modifies the activity of HVA channels as well7. Originally, Kurotoxin was shown to affect NaV channels, highlighting the structural similarities between LVA CaV channels and NaV channels6. Recently, two groups discovered additional peptidyl toxins that block NaVand T-type channels. Kurotoxin like I and II (KLI and KLII) were isolated from a scorpion venom8 and ProTx I and ProTx II from Taranula venom9.
A New Antibody to Cav3.1 (α1G subunit)
The CaV3.1 antibody was raised against a peptide MDEEEDGAGAEESGQPRSFTQL(C), corresponding to residues 1-22 of rat CaV3.1 and affinity purified on immobilized antigen. The epitope was chosen according to the article published by Andreasen et al. in which the localization of α1G in the apical membranes of the collecting tubule, distal convoluted tubule and inner medullary connecting duct of microdissected rat nephron segments was demon strated by RT-PCR and immunohistochemistry10.
The reactivity of the antibody produced in our laboratories was confirmed by western blotting with rat brain membranes.
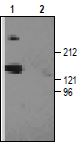
1. Anti-CACNA1G (CaV3.1) Antibody (#ACC-021) (1:200).2. Anti-CACNA1G (CaV3.1) Antibody, preincubated with the negative control antigen.
References
- Hille, B. (2001) Ion Channels of Excitable Membranes. 3rd edition.
- Perez-Reyes, E. (2003) Physiol. Rev. 83, 117.
- Wain, H.M. et al. (2002) Nucleic Acids Res. 30, 169.
- HGNC, Department of Biology, University College London (http://www.gene.ucl.ac.uk/cgibin/nomenclature/searchgenes.pl). January 2003.
- Santi, C.M. et al. (2002) J. Neurosci. 22, 396.
- Chuang, R.S.I. et al. (1998) Nat. Neurosci. 8, 668.
- Sidach, S.S. and Mintz, I.M. (2002) J. Neurosci. 22, 2023.
- Olamendi-Portugal, T. et al. (2002) Biochem. Biophys. Res. Comm. 299, 562.
- Middleton, R.E. et al. (2002) Biochemistry 41, 14734.
- Andreasen, D. et al. (2000) Am. J. Physiol. 279, F997.