In the depths of your inner ear, tiny cells are busy converting the vibrations of sound into electrical signals that your brain can interpret. These auditory inner hair cells (IHCs) perform this task with remarkable precision and sensitivity. But how exactly do they manage to translate the cacophony of noises in our environment into discernible, meaningful sounds? New research has unveiled the surprising role of potassium (K+) channels in this intricate process, demonstrating the exquisite sensitivity and dynamic range of sound detection.
Translating Sounds
The world of sound is awash with varied frequencies and intensities. Mammalian inner ears, home to the IHCs, possess the remarkable capability to process and interpret this wide spectrum. This ability is made possible by converting a tonotopic sound-frequency map into electrical signals in IHCs and outer hair cells (OHCs). These cells then give rise to depolarizing and hyperpolarizing receptor potentials. In IHCs, these potentials activate voltage-gated Ca2+ channels that trigger neurotransmitter release and set off action potential firing in auditory neurons.
Along the cochlear axis, receptor potential characteristics differ significantly. It’s as if different regions of the cochlea ‘specialize’ in encoding different aspects of sound: some are better at following frequency, others at tracking intensity. These gradations in tuning are achieved through distinct combinations of three voltage-dependent potassium currents. This process, although well-established, leaves one question unanswered: how do these IHCs manage to encode such a complex soundscape?
Probing Potassium Channels
A recent collaboration between German and Australian researchers aimed to address this auditory mystery. The team discovered that IHCs express an exclusive suite of six voltage-dependent potassium conductances, each regulated by different channels. These channels are all active at rest, but their activity can vary greatly during sound stimulation. This variability enables a kind of non-saturating tuning, allowing IHCs to respond across a vast range of potentials. Each of these conductances contributes to optimizing responses, but the combined activity of all channels synergistically enhances phase locking and the dynamic range of intensities that IHCs can encode.
The team relied on RT-PCR and fluorescent immunohistochemistry to demonstrate the expression of Kv11.1 (via #APC-016), Kv11.2 (via #APC-114), and Kv11.3 channels in cochlear lysates and cells (Figure 1).
Immunohistochemistry reveals Potassium Channel Expression
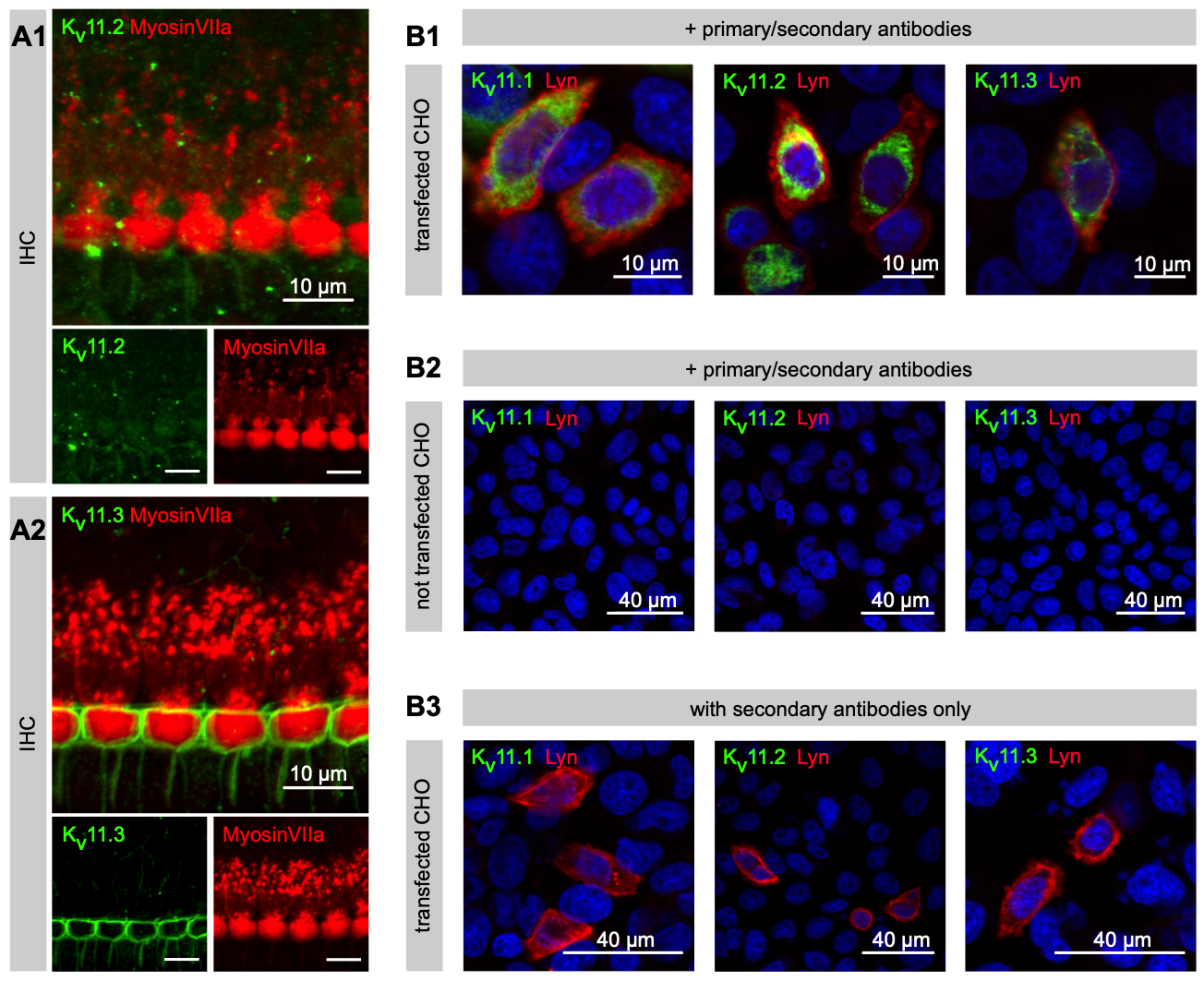
Surprisingly, the team found three previously unknown contributors to the potassium conductances in IHCs: Kv1.8, Kv11.1, and Kv12. These channels are functional in IHCs and collectively mediate a significant portion of the potassium currents, identified through their pharmacological characteristics. However, Kv3.3, another potassium channel, was not found to be present in IHCs.
While Kv12.1 currents in IHCs demonstrated a major mode shift, the researchers couldn’t find evidence for the relevance of this phenomenon for sound encoding. However, they were drawn to the idea that biophysical properties of IHCs could change given continuous sound stimulation or pathological depolarization through this mode shift, adding an extra layer of specialization to intrinsic IHC membrane tuning.
Kv7.4, another contributor to potassium conductances, mediates two distinct components in IHCs. This observation suggests that hair cell-specific interaction partners may determine the properties of Kv7.4 in IHCs. As the proportion of these conductances determines the tonotopic differences in receptor potentials, this discovery suggests an evolutionary adaptation of IHCs to encode complex sounds.
Hairy Intricacies Behind Sound
The expression of various potassium channels constitutes an important evolutionary adaptation to improve the dynamic range and temporal precision of IHC responses. These channels enable a higher-order Boltzmann nonlinearity, with steep sensitivity near rest that does not saturate over the entire range of physiological potentials. This, combined with their distinct activation during sound stimulation, allows IHCs to encode a wide array of sound intensities and frequencies, revealing an intricate, delicately balanced system of sound encoding that underscores the complexity and ingenuity of our hearing apparatus.
This study enriches our understanding of the auditory system, providing a new perspective on how ion channels enable our ears to process the rich tapestry of sounds that surround us every day. Ultimately, this insight brings us a step closer to decoding the grand symphony of sounds that our ears so beautifully translate into the world as we know it.
Antibodies
- Anti-KCNH2 (erg1) Antibody (#APC-016) (Kv11.1)
Cat #: APC-016
Type: Polyclonal | Host: Rabbit | Applications: ICC, IF, IHC, IP, WB, *IFC | Reactivity: H, M, R - Anti-KCNH2 (HERG) (extracellular) Antibody (#APC-109). This antibody recognizes a different epitope and thus can also be used as a control for detecting KV11.1 (KCNH2).
Cat #: APC-109
Type: Polyclonal | Host: Rabbit | Applications: ICC, IF, IFC, IHC, IP, LCI, WB | Reactivity: H, R, *M - Anti-KCNH2 (HERG) (extracellular)-FITC Antibody (APC-109-F). A fluorescent labeled primary antibody. It can be used to detect KV11.1 (KCNH2) in live cells
Cat #: APC-109-F
Type: Polyclonal | Host: Rabbit | Applications: FC, LCI | Reactivity: H, R - Anti-KCNH6 (erg2) Antibody (#APC-114) (Kv11.2)
Cat #: APC-114
Type: Polyclonal | Host: Rabbit | Applications: IF, IHC, WB, *ICC, *IFC, *IP | Reactivity: H, M, R - Anti-KCNH7 (erg3) Antibody (#APC-112) (Kv11.3)
Cat #: APC-112
Type: Polyclonal | Host: Rabbit | Applications: ICC, IF, IHC, WB, *IFC, *IP | Reactivity: H, M, R - Anti-KV1.8 Antibody (#APC-157)
Cat #: APC-157
Type: Polyclonal | Host: Rabbit | Applications: WB, *ICC, *IFC, *IHC, *IP | Reactivity: H, M, R - Anti-KCNQ4 Antibody (#APC-164)
Cat #: APC-164
Type: Polyclonal | Host: Rabbit | Applications: IF, IHC, WB, *ICC, *IFC, *IP | Reactivity: H, M, R - Anti-KV12.1 (KCNH8) Antibody (#APC-113)
Cat #: APC-113
Type: Polyclonal | Host: Rabbit | Applications: WB, *ICC, *IFC, *IHC, *IP | Reactivity: R, *H, *M
Pharmacological Tools
- Iberiotoxin (#STI-400) – A Potent and Specific Blocker of KCa1.1 K+ Channels
Cat #: STI-400
Type: Toxins | Source: Synthetic peptide | MW: 4230.9 Da | Target: KCa1.1 K+ channels - XE991 dihydrochloride (#X-101) – A Blocker of KCNQ Channels
Cat #: X-101
Type: Toxins | Source: Synthetic | MW: 449.37 | Target: KCNQ K+ channels - 4-Aminopyridine (#A-115) – A Non-Specific K+ Channel Blocker
Cat #: A-115
Type: Small Molecules | Source: Synthetic | MW: 94.1 | Target: KV K+ channels - NS-1643 (#N-115) – An Opener of KV11.1 and KCNQ2 K+ Channels
Cat #: N-115
Type: Small Molecules | Source: Synthetic | MW: 380.2 | Target: KV11.1, KCNQ2 K+ channels
Explorer Kits
- hERG and hERG-Related Channel Antibody Explorer Kit (#AK-222)
Cat #: AK-222 | Size: 18 Vials
- hERG Related Channel Modulator Explorer Kit (#EK-109)
Cat #: EK-109 | Size: 10 Vials