Pain perception becomes a burden and ceases to be physiologically beneficial when the threat causing the pain is gone and the pain sensations remain. Such pathological, chronic conditions appear due to improper functionality of the ion channels responsible for sensory transmission. This short piece aims at reviewing progress in nociception using Alomone Labs products.
Introduction
Ion channels responsible for pain sensation are usually located at peripheral termini of nociceptors – pain-transmitting neurons that relay information from end organs to the brain via the dorsal root ganglion. Chronic insults to the brain, which lacks nociceptors and hence is devoid of the ability to perceive the conventional sensation of pain, are manifested as migraines by specialized receptors such as subtypes of voltage-gated Ca2+ and Na+ channels, and acid sensing ion channels. Visceral pain is associated with subsets of Na+channels whereas a myriad of other membrane proteins generously expressed on muscle and skin – transient receptor potential channels, inward- and outward-rectifying K+ channels and P2X family receptors – are triggered by heat, cold, mechanical, and inflammatory stressors3,10.
NaV Channels
NaV channels are widely expressed in all excitable cells where they mediate the initiation and propagation of the action potential. NaV1.7-NaV1.9 channels are critical factors in peripheral sensation transduction. Hu et al. studied the role of these channels in the chronic pain accompanying irritable bowel syndrome (IBS) – a pathological condition of the digestive tract. To simulate IBS, they inflicted stress-induced visceral dysfunction on newborn rats by depriving them of maternal care (neonatal maternal deprivation; NMD). Following a period of seven weeks, they dissected out the deprived pups’ colon-specific dorsal root ganglia (DRG) neurons and compared them with a control group. Using Anti-NaV1.8 (SCN10A) Antibody (#ASC-016) and Anti-SCN11A (NaV1.9) Antibody (#ASC-017) in western blot analysis, a marked increase of NaV1.8 but not NaV1.9 was observed in these neurons. This finding was also confirmed by real-time PCR6. The mechanism behind the hyper sensitization of NaV1.8 – the presumed cause of the sustained pain associated with IBS – was made clear in a follow up study. Hydrogen sulfide (H2S; an endogenously produced gaseous modulator)5, produced by the enzyme cystathionine β-synthetase (CBS), significantly increases following NMD treatment. Immunohistochemical staining and western blot analysis of rat colon DRGs using Anti-NaV1.8 (SCN10A) Antibody show that the channel’s expression increases and colocalizes with CBS7. The molecular mechanism is suggested to be suppression of KV channels, namely KV1.1 and KV1.4 which also colocalize with CBS as shown in immunohistochemical staining of rat trigeminal ganglion neurons using Anti-KV1.1 (KCNA1) Antibody (#APC-009) and Anti-KV1.4 Antibody (#APC-007).
The roles of NaV channels were also studies following an analgesic treatment aimed at reducing inflammatory pain. The therapeutic technique involves needle-based electrical stimulation of muscle fibers (electroacupuncture). DRGs were dissected from mice (treated and untreated with electro-acupuncture, following inflammatory pain induction). Anti-NaV1.7 (SCN9A) Antibody (#ASC-008), Anti-NaV1.8 (SCN10A) Antibody and Anti-SCN11A (NaV1.9) Antibody were used in immunohistochemistry and immunoblotting assays to show overexpression of NaV1.7 and NaV1.8 following pain induction and their subsequent decrease after electro-acupuncture. Expression of NaV1.9 was not affected. Thus, electro-acupuncture as a means for inflammatory pain alleviation acts in part by reducing NaV1.7 and NaV1.8 expression8.
K+ Channels
Inward rectifying K+ (Kir) channels play a distinctive role in a unique population of pacemaker neurons of the developing superficial dorsal horn (SDH), as was demonstrated by Jie Li’s team. The population in question constitutes almost a third of the lamina I cells; lamina I is crucial for transmitting pain sensation to the brain, and receives its input from peripheral nociceptors. Immunohistochemistry of rat SDH using Anti-Kir2.1 (KCNJ2) Antibody (#APC-026), and Anti-Kir2.3 (KCNJ4) Antibody (#APC-032) showed that both channels are abundantly expressed in lamina I pacemaker cells (Figure 1), a result indicating the major influence these channels have on developing pain trajectories9.
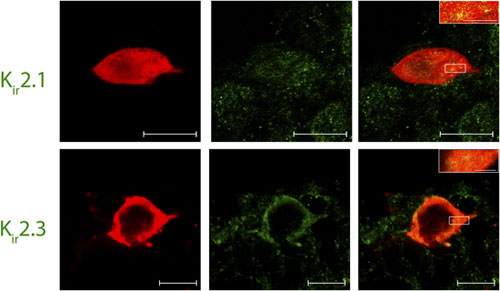
Immunohistochemical staining of rat spinal cord sections using Lamina I pacemaker using Anti-Kir2.1 (KCNJ2) Antibody (#APC-026) and Anti-Kir2.3 (KCNJ4) Antibody (#APC-032). Confocal images of representative biocytin-filled pacemaker neurons (red) processed for Kir (green). Merged images demonstrate that immunoreactive puncta for Kir2.1 and Kir2.3 (yellow; inset) are localized to identified pacemaker neurons within lamina I of the neonatal spinal cord (right). Scale bars: 10 μm; inset, 2 μm.
Adapted from reference 9 with permission of The Society for Neuroscience.
TRPV Channels
Transient receptor potential vanilloid (TRPV) channels are non-selective Ca2+ channels, broadly expressed in nociceptors where they modulate Ca2+ homeostasis and sensation stimuli. Their contribution to sensory hypersensitivity in spinal cord injured rats was explored. One month following spinal cord injury, western blot analysis of lumbar DRG neurons using Anti-Rat TRPV1 (VR1) (extracellular) Antibody (#ACC-029) showed a pronounced overexpression of TRPV1 even long after the injury. TRPV1 knock-out mice, on the other hand, had a higher sensitivity threshold, evaluated by low hind paw withdrawals scores in response to mechanical and thermal challenges11. Although continuous application of capsaicin induces TRPV1 desensitization, anchoring adenylyl cyclase to a complex formed by scaffolding proteins and TRPV1 elevates the concentration of cyclic AMP, which increases the sensitivity of the channel to inflammation inducing factors by ~100 fold in DRG neurons. Western blot analysis of immunoprecipitated DRG lysates using Anti-TRPV1 antibody shows that the vanilloid receptor is part of the complex4.
Mastication pain often stems from mechanical allodynia (over-reaction to marginal stimuli) of the mandibular joints, a debilitating morbidity that consequently reduces food consumption of patients and risks their overall well-being. Temperomandibular joint disorder (TMJD) arises due to chronic inflammation of neuronal trajectories which innervate muscles of the jawbone, and is highly prevalent in the general population. The mechanisms that give rise to the painful sensation associated with the disorder include an increase in TRPV4 expression as shown in western blot analysis of mouse trigeminal neuron lysates from TMJD model using Anti-TRPV4 Antibody (#ACC-034). TRPV4 expression increased following inflammation induction in the model mice neurons. The specificity of the antibody was tested in TRPV4 knockout mice2.
P2X7 Receptors
Pro-inflammatory cytokines are secreted in the central nervous system by microglia. P2X7 receptors are highly expressed in membranes of microglia where they mediate the immune response in the brain and spinal cord and underlie inflammatory and neuropathic pain. Although the receptors are known to permit the passage of small inorganic ions, their permeability to molecules exceeding one nanometer has been a matter of debate. Observations of large organic dyes uptake upon activation of P2X7 has been attributed to a mechanism indirectly related to P2X7. Mutagenesis of positively charged side chains of the P2X7 pore forming subunit, thereby enabling the open state of the channel allowed entrance of large molecules directly through the channel. Anti-P2X7 Receptor Antibody (#APR-004) in cell surface biotinylation immunoblot assay was used to confirm the presence of the mutated P2X7 receptor1. This study sheds some light regarding the widespread expression of P2X7 receptors in microglia.
ASICs
A possible mechanism to chronic headaches (migraines) has been suggested to involve acid sensing ion channels (ASICs), in particular, ASIC3. ASIC3 are pH sensitive cation channels and exist in both a homomeric and a heteromeric form. They are ubiquitously expressed in sensory afferents of internal tissues from which they convey painful sensations and are also expressed in the majority of dural afferent neurons. ASIC3 was shown to be sensitive to minute drops in pH levels, induced by mast cells proximate to blood vessels that surround the dural afferents of the trigeminal ganglion (TG) neurons during inflammation. Addition of APETx2 (#STA-160), an ASIC3 blocker, abolished the associated migraine symptoms in rat models. Anti-ASIC3 Antibody (#ASC-018) was used in immunohistochemical assays to confirm the expression of ASIC3 in approximately 80% of retrogradely-labeled duraoriginated TG neurons12.
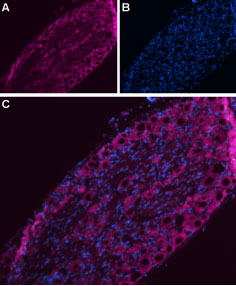
Immunohistochemical staining of rat dorsal root ganglion (DRG) using Anti-NaV1.7 (SCN9A)- ATTO-633 Antibody (#ASC-008-FR). A. NaV1.7 staining (purple) appears in DRG neurons. B. Nuclear staining using DAPI as the counterstain. C. Merge images of A and B.
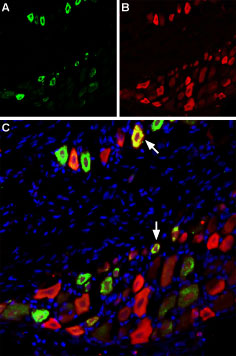
Immunohistochemical of TRPV1 and NaV1.8 in rat dorsal root ganglion using Anti-Rat TRPV1 (VR1) (extracellular)-ATTO Fluor-488 Antibody (#ACC-029-AG), (green), (1:60) and Anti-NaV1.8 (SCN10A)-ATTO Fluor-594 Antibody (#ASC-016-AR), (red), (1:60). A. TRPV1 staining. B. NaV1.8 staining. C. Merge of A and B demonstrates partial co-localization of TRPV1 and NaV1.8 channels. Nuclei stained using DAPI as the counterstain (blue).
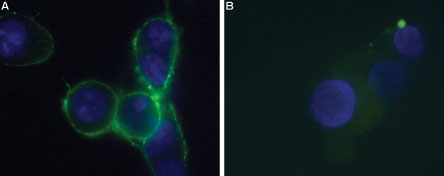
Immunocytochemical staining of live intact P2X7-HEK-293 transfected cells. A. Extracellular staining of P2X7-HEK-293 transfected cells using Anti-P2X7 (extracellular)-ATTO Fluor-488 Antibody (#APR-008-AG), (green, 1:25). B. Non-transfected HEK-293 cells also labeled with the antibody. Nucleus was stained using DAPI as the counterstain (blue).
References
- Browne, L.E. et al. (2013) J. Neurosci. 33, 3557.
- Chen, Y. et al. (2013) Pain 154, 1295.
- Cregg, R. et al. (2010) J. Physiol. 588, 1897.
- Efendiev, R. et al. (2013) J. Biol. Chem. 288, 3929.
- Feng, X. et al. (2013) Mol. Pain 9, 4.
- Hu, S. et al. (2013) Am. J. Physiol. 304, G311.
- Hu, S. et al. (2013) Exp. Neurol. 248, 275.
- Huang, C.P. et al. (2013) Evid. Based Complement Alternat. Med. 2013, 312184.
- Li, J. et al. (2013) J. Neurosci. 33, 3352.
- Raouf, R. et al. (2010) J. Clin. Invest. 120, 3745.
- Wu, Z. et al. (2013) Pain 154, 2130.
- Yan, J. et al. (2013) Headache 53, 1250.